Inmunotoxicidad y daño lisosomal en la ostra Pinctada imbricata (Röding 1758) expuesta a lubricantes usados de motores de automóviles
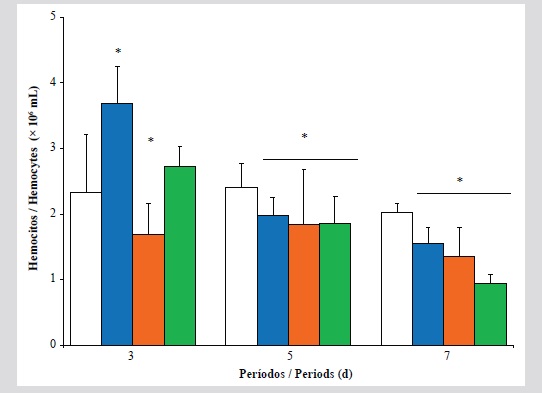
Publicado 2024-01-01
Palabras clave
- Hemocito; desestabilidad lisosomal; aceite; inmunología; estrés
Cómo citar
Derechos de autor 2023 Edgar Alexander Zapata Vivenes, Gabreial Sanchez, Leida Marcano

Esta obra está bajo una licencia internacional Creative Commons Atribución-NoComercial-CompartirIgual 4.0.
Resumen
En este estudio se evaluaron los efectos que ocasiona la exposición a fracciones acuosas de lubricantes usados de motores de automóviles (Faluma) sobre parámetros hematológicos, sistema inmune, estabilidad de la membrana lisosomal de hemocitos y niveles peroxidación
lipídica y lisozimas en la glándula digestiva de la ostra perla Pinctada imbricada. Las ostras fueron expuestas a 0, 1, 10 y 20 % v/v de Faluma durante diferentes períodos: 3, 5 y 7 días, en sistema estático de acuarios bajo condiciones controladas (oxigenación 6 mg/L; 25 ± 1 °C; pH 8.0 y 36 ‰). Durante el período temprano de exposición fue observado un aumento en el número total de hemocitos (NTH) de ostras expuestas a 10 % de Faluma. En ostras expuestas a 20 % al séptimo día se observó un descenso en los parámetros inmunohematológicos, asociados a una elevada desestabilización de la membrana lisosomal y contenido de malondialdehido (MDA). Las ostras mostraron respuestas celulares compensatorias a bajas concentraciones de Faluma, resultando mermadas durante la exposición aguda. Se evidenciaron efectos immunomodulatorios inducidos por la mezcla de compuestos xenobióticos contentivos en Faluma. Las respuestas moleculares e inmunecelulares estimadas en P. imbricada pueden suministrar información sobre los cambios en la fisiología normal de organismos que habitan
ambientes impactados por mezclas complejas de xenobióticos.
Descargas
Citas
- Aliko, V., G. Hajdaraj, A. Caci and C. Faggio. 2015. Copper induced lysosomal membrane destabilisation in haemolymph cells of mediterranean green
- crab (Carcinus aestuarii, Nardo, 1847) from the Narta Lagoon (Albania). Braz. Arch. Biol. Technol. 58(5):750–756. https://doi.org/10.1590/s1516-
- Allam, B. and D. Raftos. 2015. Immune responses to infectious diseases in bivalves. J. Invert. Pathol., 131: 121–136. https://doi.org/10.1016/j.jip.2015.05.005
- Auguste, M., Balbi, T., Ciacci, C., Canonico, B., Papa, S., Borello, A., Vezzulli, L., Canesi, L. 2020. Shift in immune parameters after repeated exposure to
- nanoplastics in the marine bivalve Mytilus. Front Immunol. 15: 11:426. https://doi.org/10.3389/fimmu.2020.00426
- Auguste, M., T. Balbi, C. Ciacci, B. Canonico, S. Papa, A. Borello, L. Vezzulli, L. Canesi, L. 2020. Shift in immune parameters after repeated exposure to
- nanoplastics in the marine bivalve Mytilus. Front Immunol. 15: 11:426. https://doi.org/10.3389/fimmu.2020.00426
- Bachère, E., R.D. Rosa, P.M. Schmitt, A. Poirier and N. Merou. 2015. The new insights into the oyster antimicrobial defense: cellular, molecular and genetic
- view. Fish and Shellfish Immunology, 2015, 46 (1), pp.50-64. https://doi.org/10.1016/j.fsi.2015.02.040
- Balbi, T., M. Auguste, C. Ciacci and L. Canesi. 2021. Immunological Responses of Marine Bivalves to Contaminant Exposure: Contribution of the -Omics
- Approach. Front Immunol. 12: 618726. https://doi.org/10.3389/fimmu.2021.618726
- Basria, S.M.N., R.I. Mydin and S. Okekpa. 2019. Reactive oxygen species, cellular redox homeostasis and cancer. homeostasis–an integrated vision. In:
- Lasacosvitsch F, S. Dos Anjos Garnes (Eds) BiotechOpen, London. https://doi.org/10.5772/intechopen.76096
- Burgos-Aceves, M. A. and C. Faggio. 2017. An approach to the study of the immunity functions of bivalve haemocytes: Physiology and molecular aspects.
- Fish & Shellfish Immunology, 67, 513–517. https://doi.org/10.1016/j.fsi.2017.06.042
- Cvengros, J., T. Liptaj and N. Pónayová. 2017. Study of polyaromatic hydrocarbons in current used motor oils, Int. J. Petrochem. Sci. Eng., 2(7) 219-226.
- https://doi.org/10.15406/ipcse.2017.02.00060
- Freitas, J.S., T.S. Boscolo-Pereira, C.N. Pereira-Boscolo, M. Navarro-García, C.A. de Oliveira-Rivero and E.A. De Almeida. 2020. Oxidative stress,
- biotransformation enzymes and histopathological alterations in Nile tilapia (Orechromis niloticus) exposed to new and used automotive lubricant oil.
- Comp. Physiol., 234: 1-11. https://doi.org/10.1016/j.cbpc.2020.108770
- Goven, A. and J. Kennedy. 1996. Environmental pollution and toxicity in invertebrates: An earthworm model for immunotoxicology. Adv. Comp. Environ.
- Physiol., 24: 170-211. https://doi.org/10.1007/978-3-642-79847-4_7
- He, L., T. He, S. Farrar, L. Ji, T. Liu and X. Ma. 2017. Antioxidants maintain cellular redox homeostasis by elimination of reactive oxygen species. Cell
- Physiol. Biochem., 44: 532-553. https://doi.org/10.1159/000485089
- Hwang, H.M., B. Stanton, T. Mcbride and M. Anderson. 2014. Polycyclic aromatic hydrocarbon body residues and lysosomal membrane destabilization
- in mussels exposed to the Dubai Star bunker fuel oil (intermediate fuel oil 380) spill in San Francisco Bay. Environ. Toxicol. Chem., 33: 1117–1121.
- https://doi.org/10.1002/etc.2518
- Jiang Y, Tang X, Sun T, Wang and Y. BDE-47 Exposure Changed the Immune Function of Haemocytes in Mytilus edulis: An Explanation Based on ROSMediated
- Pathway. Aquat Toxicol (2017) 182:58–66. https://doi.org/10.1016/j.aquatox.2016.11.010
- Liao, Y., C. Cai, C. Yang, Z. Zheng, Q. Wang, X. Du and Y. Deng. 2020. Effect of protein sources in formulated diets on the growth, immune response, and
- intestinal microflora of pearl oyster Pinctada fucata martensii. Aquac. Rep., 16: 100253. https://doi.org/10.1016/j.aqrep.2019.100253
- Lodeiros, C.J., L. Freites, A. Márquez, M.E. Glem, M. Guevara and P.E. Saucedo. 2016. Comparative growth and survival of spat of the Caribbean pearl oyster,
- Pinctada imbricata cultivated indoor with microalgae diets and outdoor with natural diet. Aquacul. Nutr., 23(3): 511–522. https://doi.org/10.1111/anu.12419
- López-Landavery, E.A., G. Amador-Cano, M.A. Tripp-Valdez, N. Ramírez-Álvarez, F. Cicala, R.J.E. Gómez-Reyes, F. Díaz, A.D. Re-Araujo and C.E.
- Galindo-Sánchez. 2022. Hydrocarbon exposure effect on energetic metabolism and immune response in Crassostrea virginica. Marine Pollution Bulletin.
- :113738. https://doi.org/10.1016/j.marpolbul.2022.113738.
- Lowe, D., M. Moore and B. Evans. 1992. Contaminant impact of interactions of molecular probes with lysosomes in living hepatocytes from dab Limanda
- limanda. Mar. Ecol. Prog. Ser., 91 (1): 135-140. https://doi.org/10.3354/meps091135
- Lowry, O., N. Rosebroungh, A. Farr and R. Randall. 1951. Protein measurement with the folin reagent. J. Biol. Chem., 193: 265-275.
- Mansour, C., F.B. Taheur and R. Omrani. 2020. Immune biomarker and hydrocarbon concentrations in carpet shell clams (Ruditapes decussatus) collected
- from a Mediterranean coastal lagoon. Euro-Mediterr J. Environ. Integr., 5: 11. https://doi.org/10.1007/s41207-020-0147-4
- Martínez-Gómez, C., J. Benedicto, J.A. Campillo and M. Moore. 2008. Application and evaluation of the neutral red retention (NRR) assay for lysosomal
- stability in mussel populations along the Iberian Mediterranean coast. J. Environ. Monit., 10(4): 490. https://doi.org/10.1039/b800441m
- Matozzo, V., M. Giacomazzo, L. Finos, M.G. Marin, L. Bargelloni and M. Milan. 2013. Can ecological history influence immunomarker responses and
- antioxidant enzyme activities in bivalves that have been experimentally exposed to contaminants? A new subject for discussion in “eco-immunology”
- studies. Fish Shell. Immunol., 35(1): 126–135. https://doi.org/10.1016/j.fsi.2013.04.013
- Méthé, D., L.A. Comeau, H. Stryhn, J.F. Burka, T. Landry and J. Davidson. 2017. Haemolymph fluid osmolality influences the neutral-red retention assay
- in the eastern oyster Crassostrea virginica, J. Molluscan Stud. 83: 229–234. https://doi.org/10.1093/mollus/eyw050
- Nusetti, O., L. Marcano, E. Zapata, M. Escalpés, S. Nusetti y C. Lodeiros. 2004. Respuestas inmunológicas y de enzimas antioxidantes en la ostra perla Pinctada
- imbricata (Mollusca: Pteridae) expuesta a niveles subletales de fuel oil Nº6. Interciencia, 29(6): 324-328. http://ve.scielo.org/scielo.php?script=sci_
- arttextypid=S0378-18442004000600008ylng=esynrm=iso
- Ohkawa, H., N. Ohishi and K. Yagi. 1979. Assay for lipid peroxides in animal tissues by thiobarbituric acid reaction, Rev. Anal. Biochem. 95: 351–358.
- https:// doi. org/10.1016/0003-2697(79)90738
- Olonisakin, A., A. Adebayo and M.O. Aremu. 2005. Metal concentrations of fresh, used and treated crankcase oil. Biosci. Biotech. Res. Asia; 3: 187-191.
- Available from: http://www.biotech-asia.org/?p=4361
- Parisi, M.G., J. Pirrera, C.M. La Corte, D. Dara, M. Parrinello and Cammarata. 2021. Effects of organic mercury on Mytilus galloprovincialis hemocyte
- function and morphology. J. Comp. Physiol. B; 191: 143–158. https://doi.org/10.1007/s00360-020-01306-0
- Renault, T. 2015. Immunotoxicological effects of environmental contaminants on marine bivalves. Fish Shellfish Immunol., 46(1): 88–93. https://doi.
- org/10.1016/j.fsi.2015.04.011
- Romero-Fereira, P., D. Arrieche, V. Acosta, L. Pérez and C. Lodeiros. 2017. Gametogenic cycle of the oyster, Pinctada imbricata, in suspended culture in
- the Gulf of Cariaco, Venezuela. Lat. Am. J. Aquat. Res.;45(1): 139-148. https://doi.org/10.3856/vol45-issue1-fulltext-13
- Sun, S., W. Shi, Y. Tang, Y. Han, X. Du, W. Zhou, Y. Hu, C. Zhou and G. Liu. 2020. Immunotoxicity of petroleum hydrocarbons and microplastics alone
- or in combination to a bivalve species: Synergic impacts and potential toxication mechanisms. Sci. Total Environm., 728: https://doi.org/10.1016/j.
- scitotenv.2020.138852
- Strober, W. 2015. Trypan blue exclusion test of cell viability. Curr. Protoc. Im. 2 111, https://doi.org/10.1002/0471142735.ima03bs111
- Sokal, R. and F. Rohlf. 2012. Biometry. 4th Ed. W.H. Freeman. New York.
- Trivedi, P.C., J.J. Bartlett and T. Pulinilkunnil. 2020. Lysosomal biology and function: Modern view of cellular debris bin. Cells, 9(5): 1131. https://
- doi. org/10.3390/cells9051131
- Vásquez, G., R. Crescini, W. Villalba, J. Mogollón y L. Troccoli. 2015. Aspectos biológicos básicos de Pinctada imbricata (Bivalvia: Pteriidae) en la laguna
- de La Restinga, isla de Margarita, Venezuela. Rev, Cienc. Mar. Cost.,7: 117-132. https://doi.org/10.15359/revmar.7.8
- Villegas, L., C. Lodeiros, K. Malavé, J. Revilla y M. Lemus. 2015. Efecto subletal del cadmio en la ostra perla del Caribe Pinctada imbricata (Pteroida:
- Pteriidae) Röding, 1798. Saber; 27 (1): 39-45
- Week, J., V. Sharp and T. Williams. 1997. Contaminant-induced lisosomal membrane damage in blood cells of green mussel Perna viridis (Mytilidae): a
- field transplant study. Technical Report WC/97/64. DFID-TDR Proyect R6191. Land-derived contaminant influx to Jakarta Bay, Indonesia; 2: 1-30.
- Wei J., B Liu, S Fan, B Zhang, J Su and D. Yu. 2017. Serum immune response of pearl oyster Pinctada fucata to xenografts and allografts. Fish Shellfish
- Immunol., 62: 303-310. https://doi.org/10.1016/j.fsi.2017.01.039
- Xie, J., C. Zhao, Q. Han, H. Zhou, Q. Li and X. Diao. 2017. Effects of pyrene exposure on immune response and oxidative stress in the pearl oyster, Pinctada
- martensii. Fish Shellfish Immunol., 63: 237–244. https://doi.org/10.1016/j.fsi.2017.02.032
- Zha, S., J. Rong, X. Guan, Y. Tang, Y. Han and G. Liu. 2019. Immunotoxicity of four nanoparticles to a marine bivalve species, Tegillarca granosa. J. Hazard
- Mater 377:237–48. https://doi.org/10.1016/j.jhazmat.2019.05.071
- Zannella, C., F. Mosca, F. Mariani, G. Franci, V. Folliero, M. Galdiero, P.G. Tiscar and M. Galdiero. 2017. Microbial diseases of bivalve mollusks: infections,
- immunology and antimicrobial defense. Mar. Drugs; 15(6):182. https://doi.org/10.3390/md15060182
- Zapata-Vívenes, E., L. Marcano y V. Acosta 2018. Respuestas inmunológicas, estabilidad lisosomal y frecuencia de micronúcleos en Eurythoe
- complanata (Polychaeta:Amphinomidae) expuestos a una fracción acuosa de lubricantes usados de motores de automóviles. Rev. Intern. Contam.
- Amb., 34 (2): 297-305. https://doi.org/10.20937/RICA.2018.34.02.10
- Zapata-Vívenes, E., O. Nusetti, L. Marcano, G. Sánchez and H. Guderley. 2020. Antioxidant defenses of flame scallop Ctenoides scaber (Born, 1778) exposed
- to the water-soluble fraction of used vehicle crankcase oils. Toxicol. Rep., 7:1597–1606. https://doi.org/10.1016/j.toxrep.2020.11.009
- Zapata Vívenes, E., G. Sánchez, O. Nusetti and L. Marcano. 2022. Modulation of innate immune responses in the flame scallop Ctenoides scaber (Born,
- caused by exposure to used automobile crankcase oils, Fish & Shellfish Immunology, 130: 342- 349. https://doi.org/10.1016/j.fsi.2022.09.020
- Zhao, C., L. Xiaoxu, L. Shibin and Y. Chang. 2011. Assessments of lysosomal membrane responses to stresses with neutral red retention assay and its potential
- application in the improvement of bivalve aquaculture. Afr, J. Biotechnol., 10 (64): 13968- 3973. https://doi.org/10.5897/AJB10.2283
- Zheng, F., F. Marques Gonçalves, Y. Abiko, H. Li, Y. Kumagai and M. Aschner. 2020. Redox toxicology of environmental chemicals causing oxidative stress.
- Redox Biol., 34: https://doi.org/10.1016/j.redox.2020.101475